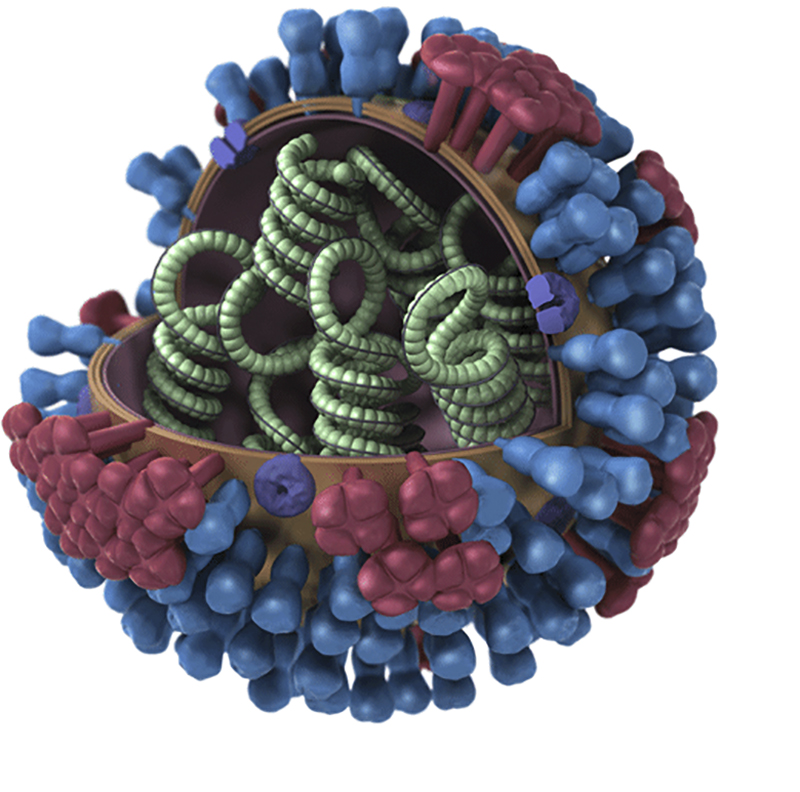
There is a very high probability that at least one of the many COVID-19 vaccine candidates out there will be successful and help humanity achieve the protection it needs from SARS-CoV-2.
It probably will not happen this year then there is still a lot of work to be done by all the teams involved and the 7 months left will probably not be enough.
A lot of doubters look at the fact that RNA viruses are tough to make vaccines for but we have them – yellow fever, measles, and polio are a few examples. Others look at how difficult it is to make a vaccine against the influenza A and B viruses. That is true but a study of the structure and properties of the flu virus explains why. A comparison of the flu virus with the SARS-CoV-2 virus exposes why we all should be hopeful that an effective vaccine for the latter is possible.
Interestingly, I think the technologies and thought processes driving the development of a COVID-19 vaccine might give us a much better flu vaccine.
One fact that needs to be emphasized over and over again is that COVID-19 is not Influenza. They may share symptoms and some pathology but they are caused by different viruses, have different contagiousness, fatality rates, and clinical pictures.
Both are RNA viruses. Where SARS-CoV-2 is part of the Beta-coronavirus family, the influenza viruses belong to the Orthomyxoviridae family.
The COVID-19 virus is made up of a single strand of RNA. That of the flu is made up of a single strand to but that strand has 8 segments. That is one of the important differences.
Though there are four types of influenza viruses: A, B, C, and D, only Types A and B cause the outbreaks of disease in humans yearly. Flu A is the most dominant and virulent type and the only type that can cause pandemics.
The viral genome of flu A codes for about 14 proteins. Two of these are hemagglutinin (HA) and neuraminidase (N). Hemagglutinin is more numerous than neuraminidase. The two are very important glycoproteins because they are integral to how the virus enters the cell. HA binds to cells of the nasal epithelium that express sialic acid. Once in the cell, the viral genome replicates. It is at this point that neuraminidase facilitates the release of the virions.
Hemagglutinin and neuraminidase are also used to name the different subtypes of the virus.
Originating mainly from birds, there are 18 subtypes of hemagglutinin and 11 subtypes of neuraminidase. Types H1 to H16 and N1 to N9 are found in birds. Types H17 – H18 and N10- N11 were isolated from bats recently. While there are potentially 198 different Influenza A subtype combinations, only 131 subtypes have been detected in nature.
The subtypes currently found in humans are H1N1(the Spanish Flu) and H3N2 (Hong Kong flu). Rarely, H1N2 has been seen. H2N2 was the cause of the Asian flu pandemic in 1957 – 58 and the subtype that caused the Spanish Flu is H1N1.
Flu B is only found in humans. There are 2 subtypes. It also has the hemagglutinin and neuraminidase glycoprotein structures too.
The genome of SARS-CoV-2 is much larger than that of the flu. Where the flu A has 13,588 bases altogether in the 8 segments, SARS-CoV-2 has about 30,000. Both viruses need an RNA polymerase for replication. One hallmark of RNA viruses is how often replication leads to mutations. Whereas the SARS-CoV-2 virus has a proofreader that can excise out mutations in the replicated genome, the flu virus does not have that capability.
Thus flu viruses show a high incidence of mutations, about 50 a year. None of these are proofread and repaired. Moreover, the virus has the ability to exchange pieces of genome between the 8 segments. Also, when two flu A types are present at the same time in a host, they can switch out gene segments, producing a new type. This phenomenon is known as reassortment and is how H1N2 came to be. It resulted from reassortment event between circulating human seasonal influenza A(H1N1) and influenza A(H3N2) viruses.
Thus the high rate of mutations, the ability to exchange genes between the segments (a process called antigen drift), and reassortment lead to an ever-changing structure of the glycoproteins not only in the virus but more importantly, on the viral envelope. The viral hemagglutinin and neuraminidase do not only allow the virus to bind to and enter the cell but they also are the antigens used for Influenza vaccines. This ability to constantly change its genetic identity leads to the vaccines that have very low efficacy, year after year.
The SARS-CoV-2 virus on the other hand does not mutate as much. The vaccine candidates against the virus target the spike glycoprotein on the envelope that has been stable through different types of the virus. The spike protein, but for some minor changes, has stayed grossly the same through SARS-CoV, MERS-CoV, and now SARS-CoV-2. It is thought that its structure will not change significantly to make vaccines as ineffective as with the flu virus.
As mentioned earlier, due to antigen drift, flu vaccines have low efficacy and must be re-engineered annually. Yearly, a global survey has to be done for the flu A strains circulating as well as the nature of the HA antigens. A projection is then made about which strains of flu A and HA antigen types to include in a vaccine, that also contains flu B.
It is no wonder flu vaccines are sometimes only about 20% effective.
One way to improve the antibody response is to use a wide collection of influenza viruses that incorporates the most common amino acids found in hemagglutinin. This technique is known as computationally optimized broadly reactive antigen (COBRA).
Now, as mentioned earlier, the dominant glycoprotein on the envelope of the flu virus is Hemagglutinin (HA). HA is structured like a mushroom, with a stalk and head. The head has the binding sites for the virus and is the dominant antigen in the inactivated, live attenuated, and
recombinant HA vaccines. Unfortunately, it is that part of HA that sees the most mutations. However the stalk, like the spike protein of the SARS-CoV-2 virus, has stayed conserved over the decades. In studies where it was used as the preferred antigen, it produced antibodies that are more broadly reactive.
Instead of presenting it as an inactivated or live version, the genetic material that codes for the stalk could be presented in a vector just like it is being done with COVID-19 vaccine candidates. That will also shorten production time then the present flu vaccines need embryonated eggs for production.
COVID-19 has dealt the world a terrible blow but it does have some silver linings. The use of vectors to present genetic material as a vaccine, the choice of conserved parts of the virus as antigens, and the avoidance of inactivated and attenuated viral products may not only give us more vaccine possibilities but also hasten how vaccines are produced.
One possibility is that we may finally get better and more effective Influenza vaccines.